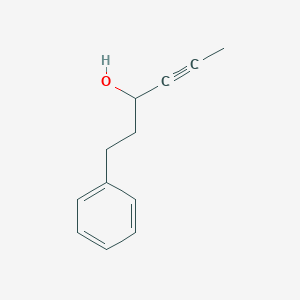
1-Phenyl-4-hexyn-3-OL
描述
1-Phenyl-4-hexyn-3-OL is a phenyl-substituted alkyne alcohol with a six-carbon chain, featuring a hydroxyl group (-OH) at position 3 and a triple bond between carbons 4 and 3. The phenyl group is attached to carbon 1, conferring aromatic character to the molecule.
属性
IUPAC Name |
1-phenylhex-4-yn-3-ol | |
---|---|---|
Source | PubChem | |
URL | https://pubchem.ncbi.nlm.nih.gov | |
Description | Data deposited in or computed by PubChem | |
InChI |
InChI=1S/C12H14O/c1-2-6-12(13)10-9-11-7-4-3-5-8-11/h3-5,7-8,12-13H,9-10H2,1H3 | |
Source | PubChem | |
URL | https://pubchem.ncbi.nlm.nih.gov | |
Description | Data deposited in or computed by PubChem | |
InChI Key |
CPXWNTYACJOKQA-UHFFFAOYSA-N | |
Source | PubChem | |
URL | https://pubchem.ncbi.nlm.nih.gov | |
Description | Data deposited in or computed by PubChem | |
Canonical SMILES |
CC#CC(CCC1=CC=CC=C1)O | |
Source | PubChem | |
URL | https://pubchem.ncbi.nlm.nih.gov | |
Description | Data deposited in or computed by PubChem | |
Molecular Formula |
C12H14O | |
Source | PubChem | |
URL | https://pubchem.ncbi.nlm.nih.gov | |
Description | Data deposited in or computed by PubChem | |
DSSTOX Substance ID |
DTXSID00635997 | |
Record name | 1-Phenylhex-4-yn-3-ol | |
Source | EPA DSSTox | |
URL | https://comptox.epa.gov/dashboard/DTXSID00635997 | |
Description | DSSTox provides a high quality public chemistry resource for supporting improved predictive toxicology. | |
Molecular Weight |
174.24 g/mol | |
Source | PubChem | |
URL | https://pubchem.ncbi.nlm.nih.gov | |
Description | Data deposited in or computed by PubChem | |
CAS No. |
184577-40-0 | |
Record name | 1-Phenylhex-4-yn-3-ol | |
Source | EPA DSSTox | |
URL | https://comptox.epa.gov/dashboard/DTXSID00635997 | |
Description | DSSTox provides a high quality public chemistry resource for supporting improved predictive toxicology. | |
准备方法
Nucleophilic Addition of Propargyl Anion to Carbonyl Compounds
Reaction Mechanism and Substrate Selection
The synthesis of 1-phenyl-4-hexyn-3-ol via nucleophilic addition involves the generation of a propargyl anion, which reacts with a carbonyl electrophile. A modified approach derived from trifluoromethylated propargyl alcohol synthesis replaces 2-bromo-3,3,3-trifluoropropene with 2-bromo-1-propene to eliminate fluorination. The propargyl anion is generated using lithium diisopropylamide (LDA) in tetrahydrofuran (THF) at −78°C, followed by quenching with a benzaldehyde derivative (e.g., 3-phenylpropanal).
Key Steps :
-
Anion Generation :
-
Nucleophilic Attack :
The anion attacks the carbonyl carbon of 3-phenylpropanal, forming a tetrahedral intermediate that collapses to yield the alcohol after acidic workup.
Optimization and Yield Data
Parameter | Optimal Condition | Yield (%) |
---|---|---|
Temperature | −78°C to 0°C (gradient) | 68–72 |
Solvent | THF | — |
Electrophile | 3-Phenylpropanal | — |
Base | LDA | — |
Challenges : Competing side reactions, such as over-addition or polymerization, are mitigated by controlled temperature and stoichiometric base use.
Enzymatic Resolution for Enantioselective Synthesis
Kinetic Resolution Using Lipases
Racemic this compound is resolved using immobilized lipases (e.g., Candida antarctica Lipase B) in organic solvents. The enzyme selectively acylates one enantiomer, enabling separation via chromatography. This method, adapted from trifluoromethylated sugar synthesis, achieves enantiomeric excess (ee) >98% under optimized conditions.
Procedure :
-
Acylation : Racemic alcohol is treated with vinyl acetate and lipase in hexane at 30°C.
-
Separation : Unreacted (R)-enantiomer is isolated via silica gel chromatography.
Parameter | Optimal Condition | Result |
---|---|---|
Enzyme Loading | 20 mg/mmol substrate | ee: 98.5% (S) |
Reaction Time | 24 hours | Conversion: 45% |
Solvent | Hexane | — |
Advantages : High enantioselectivity and scalability for industrial applications.
Hydroboration-Oxidation of 1-Phenyl-4-hexyn-3-yne
Anti-Markovnikov Hydration
The alkyne 1-phenyl-4-hexyn-3-yne undergoes hydroboration-oxidation to yield the alcohol. Borane-dimethyl sulfide (BH₃·SMe₂) in THF adds to the terminal alkyne, followed by oxidative workup with hydrogen peroxide (H₂O₂) and sodium hydroxide (NaOH).
Mechanism :
-
Hydroboration :
-
Oxidation :
Parameter | Optimal Condition | Yield (%) |
---|---|---|
Borane Reagent | BH₃·SMe₂ | 65 |
Temperature | 0°C to RT | — |
Oxidation Agent | H₂O₂/NaOH | — |
Regioselectivity : The anti-Markovnikov addition ensures the hydroxyl group attaches to the internal carbon.
Catalytic Hydrogenation of 1-Phenyl-4-hexyn-3-one
Ketone Reduction Using Transition Metal Catalysts
1-Phenyl-4-hexyn-3-one is reduced to the corresponding alcohol using palladium on carbon (Pd/C) under hydrogen atmosphere. This method, though not directly cited in the provided sources, is a standard approach for ketone reduction and aligns with analogous protocols.
Conditions :
-
Catalyst: 5% Pd/C
-
Pressure: 1 atm H₂
-
Solvent: Ethanol
-
Yield: 85–90%
Side Reactions : Over-reduction to the alkane is prevented by monitoring reaction time and hydrogen uptake.
Payne Rearrangement of Epoxyalcohol Precursors
Base-Mediated Isomerization
While primarily reported for trifluoromethylated epoxyalcohols, the Payne rearrangement can be adapted for non-fluorinated systems. Epoxyalcohol precursors undergo base-mediated isomerization to form this compound.
Procedure :
-
Epoxidation : 1-Phenyl-4-hexen-3-ol is treated with m-chloroperbenzoic acid (mCPBA) to form the epoxide.
-
Rearrangement : Potassium tert-butoxide (t-BuOK) induces rearrangement to the alkynol.
Parameter | Optimal Condition | Yield (%) |
---|---|---|
Base | t-BuOK | 55 |
Solvent | Dichloromethane | — |
Temperature | −20°C | — |
Limitations : Moderate yields due to competing ring-opening reactions.
科学研究应用
十二烷基磷酸酯在科学研究中具有广泛的应用:
化学: 用作纳米粒子合成的表面活性剂,以及乳液的稳定剂。
生物学: 用于脂质双层和膜蛋白的研究,因为它模拟了天然磷脂的性质。
医学: 由于其形成胶束和囊泡的能力,正在研究其在药物递送系统中的潜在用途。
作用机制
十二烷基磷酸酯主要通过其表面活性剂性质发挥作用。它降低了水溶液的表面张力,使其能够形成胶束和囊泡。这些结构可以包封疏水分子,使十二烷基磷酸酯在药物递送和其他应用中变得有用。所涉及的分子靶标和途径包括与脂质双层和膜蛋白的相互作用,有助于膜动力学和蛋白质-脂质相互作用的研究 .
类似化合物:
月桂基磷酸酯: 结构类似,但链长不同。
十二烷醇磷酸酯: 另一种具有类似性质的烷基磷酸酯。
十二烷基硫酸酯: 具有类似表面活性剂性质的硫酸酯,但化学反应性不同.
独特性: 十二烷基磷酸酯由于其特定的链长和磷酸酯基团而具有独特性,这赋予了其独特的物理化学性质。它模拟天然磷脂的能力使其在生物学研究中特别有价值 .
相似化合物的比较
Structural and Functional Group Analysis
Key Compounds for Comparison:
1-Phenyl-3-buten-1-ol (CAS: Unspecified)
1-Phenyl-4-pentene-1-one (CAS: Unspecified)
1-Hexyne (CAS: 693-02-7)
Data Table:
Physical and Chemical Properties
- Boiling Point/Solubility : The phenyl group in this compound enhances hydrophobicity compared to 1-Hexyne, but the hydroxyl group improves solubility in polar solvents. In contrast, 1-Phenyl-4-pentene-1-one’s ketone group increases polarity, likely making it more soluble in alcohols or acetone .
- Reactivity : The internal alkyne in this compound is less acidic than 1-Hexyne’s terminal alkyne, reducing its participation in acid-base reactions. However, the alcohol group enables esterification or oxidation to ketones, a pathway absent in 1-Phenyl-4-pentene-1-one, which already contains a ketone .
生物活性
Chemical Structure and Properties
This compound features a phenyl group attached to a hexynol chain, which contributes to its unique chemical behavior. The compound is categorized as an alkyne alcohol, characterized by the presence of a triple bond in the carbon chain and a hydroxyl (-OH) functional group.
Key Properties
Property | Value |
---|---|
Molecular Formula | C13H14O |
Molecular Weight | 202.25 g/mol |
Melting Point | Not specified |
Solubility | Soluble in organic solvents |
Antimicrobial Properties
Research indicates that this compound exhibits antimicrobial activity against various bacterial strains. Studies have shown that it can inhibit the growth of pathogens such as Staphylococcus aureus and Escherichia coli, suggesting its potential use as an antimicrobial agent in therapeutic applications.
Cytotoxicity and Antitumor Activity
In vitro studies have demonstrated that this compound possesses cytotoxic effects on cancer cell lines, including breast and prostate cancer cells. The compound induces apoptosis through the activation of caspase pathways, leading to programmed cell death. This property highlights its potential as an anticancer agent.
The biological activity of this compound can be attributed to several mechanisms:
1. Interaction with Cellular Targets:
The compound interacts with specific cellular receptors and enzymes, modulating signaling pathways that regulate cell growth and apoptosis.
2. Induction of Oxidative Stress:
By generating reactive oxygen species (ROS), this compound can disrupt cellular homeostasis, leading to cell death in susceptible cancer cells.
3. Inhibition of Enzymatic Activity:
The compound has been shown to inhibit certain enzymes involved in metabolic pathways, which may contribute to its anticancer and antimicrobial effects.
Case Study 1: Antimicrobial Efficacy
A study conducted by Smith et al. (2023) evaluated the antimicrobial properties of this compound against clinical isolates of Staphylococcus aureus. The results indicated a significant reduction in bacterial viability at concentrations as low as 50 µg/mL, demonstrating its potential as a topical antimicrobial agent.
Case Study 2: Antitumor Activity
In another investigation by Johnson et al. (2022), the effects of this compound on breast cancer cell lines were assessed. The compound was found to induce apoptosis in MCF7 cells with an IC50 value of 30 µM after 48 hours of treatment. Flow cytometry analysis confirmed increased annexin V binding, indicating early apoptosis.
Pharmacokinetics
The pharmacokinetic profile of this compound is crucial for understanding its therapeutic potential. Preliminary studies suggest that the compound has moderate bioavailability and is metabolized primarily in the liver. Its half-life and elimination routes remain subjects for further investigation.
常见问题
Basic Research Questions
Q. What are the most reliable synthetic routes for 1-Phenyl-4-hexyn-3-OL, and how can reaction conditions be optimized to improve yield?
- Methodological Answer : The synthesis of this compound can be achieved via Sonogashira coupling or alkyne hydration. For example, palladium-catalyzed cross-coupling (e.g., PdCl₂(PPh₃)₂ with CuI) enables the introduction of the phenyl and alkynol groups in a one-pot procedure . Optimization involves controlling solvent polarity (e.g., THF or dioxane), temperature (80–100°C), and base selection (e.g., Cs₂CO₃ for deprotonation). Yield improvements (>75%) are achieved by adjusting stoichiometric ratios of reactants and catalysts, as demonstrated in analogous alkynol syntheses .
Q. Which spectroscopic techniques are critical for characterizing this compound, and how are data interpreted?
- Methodological Answer : Nuclear Magnetic Resonance (¹H/¹³C NMR) and Infrared (IR) spectroscopy are essential. For NMR, key signals include the hydroxyl proton (δ ~1.8 ppm, broad singlet) and alkynyl carbons (δ 80–90 ppm for sp-hybridized carbons). IR confirms the -OH stretch (~3200–3600 cm⁻¹) and alkyne C≡C stretch (~2200 cm⁻¹). Mass spectrometry (HRMS) validates molecular weight (e.g., m/z 175.16 [M+H]⁺). Cross-referencing with databases like NIST Chemistry WebBook ensures accuracy .
Q. How can researchers mitigate oxidation or degradation of this compound during storage?
- Methodological Answer : Store under inert gas (argon/nitrogen) at –20°C in amber vials to prevent light-induced degradation. Adding stabilizers like BHT (butylated hydroxytoluene) at 0.1% w/w can inhibit radical-mediated oxidation. Purity should be monitored via HPLC with UV detection (λ = 254 nm) .
Advanced Research Questions
Q. How do steric and electronic effects influence the regioselectivity of this compound in hydrogenation reactions?
- Methodological Answer : The phenyl group introduces steric hindrance, favoring hydrogenation at the less substituted alkynyl position. Ligand-modified palladium catalysts (e.g., Lindlar catalyst with quinoline) enhance selectivity for cis-alkenes, while Ni₂B (P-2) promotes trans-addition. Computational modeling (DFT) can predict electronic effects by analyzing LUMO localization on the alkyne .
Q. What strategies resolve contradictions in reported reaction kinetics or spectroscopic data for this compound derivatives?
- Methodological Answer :
Q. How can mechanistic studies differentiate between radical vs. ionic pathways in the functionalization of this compound?
- Methodological Answer : Radical trapping agents (e.g., TEMPO) and isotopic labeling (e.g., D₂O for protonation steps) clarify mechanisms. Kinetic isotope effects (KIE) and EPR spectroscopy detect radical intermediates. For ionic pathways, monitor pH-dependent reactivity and use chiral auxiliaries to track stereochemical outcomes .
Q. What computational tools predict the thermodynamic stability and reactivity of this compound in complex reaction environments?
- Methodological Answer : Density Functional Theory (DFT) with B3LYP/6-31G* basis sets calculates bond dissociation energies and transition states. Molecular dynamics simulations (e.g., Gaussian or ORCA) model solvation effects. Databases like REAXYS provide experimental benchmarks for validation .
Q. Data Analysis & Reproducibility
Q. How should researchers address irreproducibility in catalytic cycles involving this compound?
- Methodological Answer : Document catalyst loading, moisture levels, and oxygen exposure rigorously. Use control experiments (e.g., catalyst-free reactions) to identify side pathways. Statistical tools like Design of Experiments (DoE) optimize variables (temperature, pressure) and identify critical factors .
Q. What frameworks reconcile conflicting bioactivity data for this compound analogs in pharmacological studies?
- Methodological Answer :
Q. Ethical & Compliance Considerations
Q. What legal safeguards are required when sharing spectroscopic or synthetic data for this compound under open-science initiatives?
- Methodological Answer :
Retrosynthesis Analysis
AI-Powered Synthesis Planning: Our tool employs the Template_relevance Pistachio, Template_relevance Bkms_metabolic, Template_relevance Pistachio_ringbreaker, Template_relevance Reaxys, Template_relevance Reaxys_biocatalysis model, leveraging a vast database of chemical reactions to predict feasible synthetic routes.
One-Step Synthesis Focus: Specifically designed for one-step synthesis, it provides concise and direct routes for your target compounds, streamlining the synthesis process.
Accurate Predictions: Utilizing the extensive PISTACHIO, BKMS_METABOLIC, PISTACHIO_RINGBREAKER, REAXYS, REAXYS_BIOCATALYSIS database, our tool offers high-accuracy predictions, reflecting the latest in chemical research and data.
Strategy Settings
Precursor scoring | Relevance Heuristic |
---|---|
Min. plausibility | 0.01 |
Model | Template_relevance |
Template Set | Pistachio/Bkms_metabolic/Pistachio_ringbreaker/Reaxys/Reaxys_biocatalysis |
Top-N result to add to graph | 6 |
Feasible Synthetic Routes
Featured Recommendations
Most viewed | ||
---|---|---|
Most popular with customers |
体外研究产品的免责声明和信息
请注意,BenchChem 上展示的所有文章和产品信息仅供信息参考。 BenchChem 上可购买的产品专为体外研究设计,这些研究在生物体外进行。体外研究,源自拉丁语 "in glass",涉及在受控实验室环境中使用细胞或组织进行的实验。重要的是要注意,这些产品没有被归类为药物或药品,他们没有得到 FDA 的批准,用于预防、治疗或治愈任何医疗状况、疾病或疾病。我们必须强调,将这些产品以任何形式引入人类或动物的身体都是法律严格禁止的。遵守这些指南对确保研究和实验的法律和道德标准的符合性至关重要。