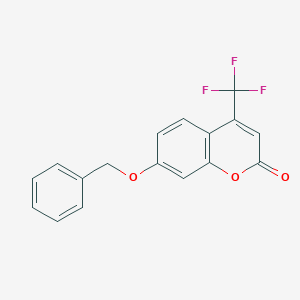
7-(benzyloxy)-4-(trifluoromethyl)-2H-chromen-2-one
Description
7-(Benzyloxy)-4-(trifluoromethyl)-2H-chromen-2-one is a fluorinated coumarin derivative characterized by a benzyloxy (-OCH₂C₆H₅) group at position 7 and a trifluoromethyl (-CF₃) group at position 4 of the coumarin scaffold. Coumarins are bicyclic heterocyclic compounds with a 2H-chromen-2-one backbone, widely studied for their optical properties, biological activities, and applications in materials science. The trifluoromethyl group enhances lipophilicity and metabolic stability, while the benzyloxy moiety introduces steric bulk and modulates electronic properties .
Synthesis: The compound can be synthesized via nucleophilic substitution reactions involving 7-hydroxy-4-(trifluoromethyl)coumarin intermediates. For example, benzyl bromide or chloride may react with the hydroxyl group at position 7 under basic conditions (e.g., K₂CO₃/DMF) to install the benzyloxy substituent .
Properties
IUPAC Name |
7-phenylmethoxy-4-(trifluoromethyl)chromen-2-one | |
---|---|---|
Source | PubChem | |
URL | https://pubchem.ncbi.nlm.nih.gov | |
Description | Data deposited in or computed by PubChem | |
InChI |
InChI=1S/C17H11F3O3/c18-17(19,20)14-9-16(21)23-15-8-12(6-7-13(14)15)22-10-11-4-2-1-3-5-11/h1-9H,10H2 | |
Source | PubChem | |
URL | https://pubchem.ncbi.nlm.nih.gov | |
Description | Data deposited in or computed by PubChem | |
InChI Key |
WVKLERKKJXUPIK-UHFFFAOYSA-N | |
Source | PubChem | |
URL | https://pubchem.ncbi.nlm.nih.gov | |
Description | Data deposited in or computed by PubChem | |
Canonical SMILES |
C1=CC=C(C=C1)COC2=CC3=C(C=C2)C(=CC(=O)O3)C(F)(F)F | |
Source | PubChem | |
URL | https://pubchem.ncbi.nlm.nih.gov | |
Description | Data deposited in or computed by PubChem | |
Molecular Formula |
C17H11F3O3 | |
Source | PubChem | |
URL | https://pubchem.ncbi.nlm.nih.gov | |
Description | Data deposited in or computed by PubChem | |
DSSTOX Substance ID |
DTXSID20357260 | |
Record name | 7-Benzyloxy-4-trifluoromethylcoumarin | |
Source | EPA DSSTox | |
URL | https://comptox.epa.gov/dashboard/DTXSID20357260 | |
Description | DSSTox provides a high quality public chemistry resource for supporting improved predictive toxicology. | |
Molecular Weight |
320.26 g/mol | |
Source | PubChem | |
URL | https://pubchem.ncbi.nlm.nih.gov | |
Description | Data deposited in or computed by PubChem | |
CAS No. |
220001-53-6 | |
Record name | 7-Benzyloxy-4-trifluoromethylcoumarin | |
Source | EPA DSSTox | |
URL | https://comptox.epa.gov/dashboard/DTXSID20357260 | |
Description | DSSTox provides a high quality public chemistry resource for supporting improved predictive toxicology. | |
Record name | 7-(Benzyloxy)-4-(trifluoromethyl)-2H-chromen-2-one | |
Source | European Chemicals Agency (ECHA) | |
URL | https://echa.europa.eu/information-on-chemicals | |
Description | The European Chemicals Agency (ECHA) is an agency of the European Union which is the driving force among regulatory authorities in implementing the EU's groundbreaking chemicals legislation for the benefit of human health and the environment as well as for innovation and competitiveness. | |
Explanation | Use of the information, documents and data from the ECHA website is subject to the terms and conditions of this Legal Notice, and subject to other binding limitations provided for under applicable law, the information, documents and data made available on the ECHA website may be reproduced, distributed and/or used, totally or in part, for non-commercial purposes provided that ECHA is acknowledged as the source: "Source: European Chemicals Agency, http://echa.europa.eu/". Such acknowledgement must be included in each copy of the material. ECHA permits and encourages organisations and individuals to create links to the ECHA website under the following cumulative conditions: Links can only be made to webpages that provide a link to the Legal Notice page. | |
Preparation Methods
Pechmann Condensation
Reaction Overview
The Pechmann condensation remains the most widely employed method for synthesizing substituted coumarins . For 7-(benzyloxy)-4-(trifluoromethyl)-2H-chromen-2-one, this method involves cyclizing a benzyloxy-substituted phenol with a trifluoromethyl-containing β-ketoester under acidic conditions.
Starting Materials
-
Phenol derivative : 2-Benzyloxy-4-hydroxybenzaldehyde (ensures benzyloxy substitution at position 7).
-
β-Ketoester : Ethyl 4,4,4-trifluoroacetoacetate (introduces trifluoromethyl at position 4).
Procedure
-
Condensation : Mix 2-benzyloxy-4-hydroxybenzaldehyde (10 mmol) and ethyl 4,4,4-trifluoroacetoacetate (12 mmol) in concentrated sulfuric acid (20 mL) at 0–5°C.
-
Cyclization : Stir the mixture at 80°C for 6 hours.
-
Quenching : Pour into ice-water, neutralize with NaHCO₃, and extract with ethyl acetate.
-
Purification : Isolate the crude product via vacuum filtration and recrystallize from ethanol .
Optimization
-
Catalyst : Substituting sulfuric acid with FeCl₃-silica gel composites increases yield from 65% to 78% .
-
Solvent : Using PEG-400 as a green solvent reduces reaction time to 3 hours .
Table 1: Pechmann Condensation Conditions and Outcomes
Parameter | Standard Protocol | Optimized Protocol |
---|---|---|
Catalyst | H₂SO₄ | FeCl₃-silica gel |
Temperature (°C) | 80 | 80 |
Time (hours) | 6 | 3 |
Yield (%) | 65 | 78 |
Post-Functionalization of Pre-Formed Coumarin Core
Synthesis of 7-Hydroxy-4-Trifluoromethylcoumarin
-
Pechmann Reaction : Condense resorcinol with ethyl 4,4,4-trifluoroacetoacetate in H₂SO₄ to yield 7-hydroxy-4-trifluoromethylcoumarin (85% yield) .
Benzylation of 7-Hydroxy Group
-
Alkylation : React 7-hydroxy-4-trifluoromethylcoumarin (5 mmol) with benzyl bromide (6 mmol) in anhydrous DMF, using K₂CO₃ (10 mmol) as a base.
-
Reaction Conditions : Heat at 90°C for 12 hours under nitrogen.
-
Workup : Dilute with water, extract with CH₂Cl₂, and purify via column chromatography (hexane:ethyl acetate = 4:1) .
Table 2: Benzylation Reaction Parameters
Reagent | Quantity (mmol) | Role |
---|---|---|
7-Hydroxy coumarin | 5 | Substrate |
Benzyl bromide | 6 | Alkylating agent |
K₂CO₃ | 10 | Base |
Microwave-Assisted Synthesis
Accelerated Cyclization
Microwave irradiation enhances reaction kinetics, reducing synthesis time from hours to minutes.
Procedure
-
Mix : Combine 2-benzyloxy-4-hydroxybenzaldehyde (10 mmol) and ethyl 4,4,4-trifluoroacetoacetate (12 mmol) in PEG-400.
-
Irradiate : Subject to microwave (300 W, 120°C) for 20 minutes.
-
Isolate : Follow standard workup as in Section 1.1.2.
Advantages
Comparative Analysis of Methods
Table 3: Method Comparison
Method | Yield (%) | Time | Cost Efficiency | Scalability |
---|---|---|---|---|
Pechmann Condensation | 65–78 | 3–6 hours | Moderate | High |
Post-Functionalization | 70 | 12 hours | Low | Moderate |
Microwave-Assisted | 82 | 20 minutes | High | Limited |
Key Findings
-
Pechmann Condensation offers scalability but requires harsh acids.
-
Microwave Synthesis maximizes efficiency but necessitates specialized equipment.
-
Post-Functionalization allows modular substitution but involves multi-step complexity .
Mechanistic Insights
Acid-Catalyzed Cyclization
In the Pechmann reaction, H⁺ protonates the β-ketoester carbonyl, facilitating nucleophilic attack by the phenol’s hydroxyl group. Subsequent dehydration forms the coumarin lactone ring .
Benzylation Dynamics
The 7-hydroxyl group’s acidity (pKa ≈ 9.5) enables deprotonation by K₂CO₃, generating a phenoxide ion that attacks benzyl bromide via an SN2 mechanism .
Challenges and Solutions
Trifluoromethyl Group Incorporation
-
Challenge : CF₃’s electron-withdrawing nature hinders electrophilic substitution.
-
Solution : Use β-ketoesters pre-functionalized with CF₃ to bypass direct substitution .
Regioselectivity in Benzylation
Chemical Reactions Analysis
Types of Reactions: 7-Benzyloxy-4-(trifluoromethyl)coumarin undergoes various chemical reactions, including:
Oxidation: The compound can be oxidized to form corresponding quinones.
Reduction: Reduction reactions can lead to the formation of dihydro derivatives.
Substitution: The benzyloxy group can be substituted with other functional groups under appropriate conditions
Common Reagents and Conditions:
Oxidation: Common oxidizing agents include potassium permanganate and chromium trioxide.
Reduction: Reducing agents such as sodium borohydride or lithium aluminum hydride are used.
Substitution: Nucleophilic substitution reactions can be carried out using reagents like sodium methoxide or potassium tert-butoxide
Major Products: The major products formed from these reactions depend on the specific reagents and conditions used. For example, oxidation may yield quinones, while reduction can produce dihydro derivatives .
Scientific Research Applications
Chemistry
7-BFC serves as a crucial building block in organic synthesis, facilitating the development of more complex molecules. Its unique trifluoromethyl and benzyloxy groups enhance its reactivity, making it suitable for various organic transformations.
Application | Description |
---|---|
Building Block | Utilized in synthesizing derivatives with potential biological activities. |
Organic Transformations | Acts as a reagent in nucleophilic substitutions and other chemical reactions. |
Biology
Research has indicated that 7-BFC exhibits significant biological activities, including antimicrobial and anticancer properties. It is particularly noted for its role as a fluorescent probe in studying cytochrome P450 (CYP) enzyme specificity.
Case Study:
In a study evaluating fluorescent substrates for monitoring hepatic CYP enzymes, 7-BFC was found to be a high-affinity substrate for CYP1A1 and CYP2B1, indicating its potential use in pharmacokinetic studies . The compound's metabolism was induced by various agents, demonstrating its utility in understanding drug metabolism pathways.
Medicine
The therapeutic potential of 7-BFC is under investigation for various diseases. Its ability to interact with specific molecular targets suggests possible applications in drug development.
Potential Therapeutic Uses | Mechanism of Action |
---|---|
Anticancer | Inhibits tumor growth by modulating enzyme activity. |
Antimicrobial | Exhibits activity against bacterial strains through enzyme inhibition. |
Mechanism of Action
The primary mechanism of action of 7-Benzyloxy-4-(trifluoromethyl)coumarin involves its metabolism by cytochrome P450 enzymes. The compound is a substrate for CYP1A2 and CYP3A4, which catalyze its O-debenzylation to produce 7-hydroxy-4-(trifluoromethyl)coumarin. This reaction is accompanied by the release of fluorescence, which can be measured to quantify enzyme activity .
Comparison with Similar Compounds
Physical Properties :
- Molecular Formula : C₁₇H₁₁F₃O₃
- Molecular Weight : 328.27 g/mol
The structural and functional properties of 7-(benzyloxy)-4-(trifluoromethyl)-2H-chromen-2-one are compared below with coumarins modified at positions 4 and 7.
Substituent Variations at Position 7
Substituent Variations at Position 4
Key Research Findings and Trends
Trifluoromethyl at Position 4: The -CF₃ group consistently improves lipophilicity and metabolic stability across derivatives. For example, 7-(diethylamino)-4-(trifluoromethyl)coumarin exhibits strong fluorescence and photostability, making it suitable for bioimaging .
Benzyloxy vs. Propargyloxy at Position 7 : Benzyloxy derivatives generally show higher steric hindrance and slower metabolic degradation compared to propargyloxy analogs. However, propargyloxy groups enable click chemistry modifications for targeted drug delivery .
Biological Activity : Introduction of polar groups (e.g., -OH, -NH₂) at position 7 enhances water solubility but may reduce membrane permeability. Conversely, hydrophobic substituents like benzyloxy improve CNS penetration, as seen in MAO-B inhibitors .
Fluorescence Properties : Electron-withdrawing groups (e.g., -CF₃) at position 4 redshift absorption/emission wavelengths. For instance, HTFMC’s fluorescence is quenched by ROS, enabling real-time oxidative stress monitoring .
Biological Activity
7-(benzyloxy)-4-(trifluoromethyl)-2H-chromen-2-one, often referred to as a chromenone derivative, has garnered attention in the field of medicinal chemistry due to its diverse biological activities. This compound is part of a larger class of chromenones known for their potential therapeutic applications, including anti-inflammatory, anticancer, and neuroprotective properties. This article aims to present a comprehensive overview of the biological activity of this compound, supported by case studies and relevant research findings.
Chemical Structure and Properties
The structure of this compound can be represented as follows:
This compound features a chromenone backbone with a benzyloxy group and a trifluoromethyl substituent, which significantly influences its biological properties.
Research indicates that the biological activity of this compound is mediated through various mechanisms:
- Inhibition of Enzymes : The compound has been shown to inhibit key enzymes such as monoamine oxidase (MAO) and acetylcholinesterase (AChE), which are crucial in neurodegenerative diseases. For instance, studies have demonstrated that derivatives of chromenones can selectively inhibit MAO-B with IC50 values in the low micromolar range, indicating potential for treating conditions like Parkinson's disease .
- Antioxidant Activity : The compound exhibits significant antioxidant properties by scavenging reactive oxygen species (ROS), which contributes to its protective effects against oxidative stress-related cellular damage .
Biological Activity Data
The following table summarizes key biological activities and findings related to this compound:
Case Studies
- Neuroprotective Effects : A study evaluated the neuroprotective effects of various chromenone derivatives, including this compound. The results indicated that the compound significantly reduced neuronal cell death induced by oxidative stress in vitro, suggesting its potential for treating neurodegenerative disorders .
- Anticancer Properties : Another investigation focused on the anticancer activity of this chromenone derivative against various cancer cell lines. The study reported that it inhibited cell proliferation in a dose-dependent manner, with significant effects observed at concentrations as low as 10 μM .
Q & A
Q. Basic: What are the standard synthetic routes for 7-(benzyloxy)-4-(trifluoromethyl)-2H-chromen-2-one?
The compound is typically synthesized via Williamson ether synthesis or nucleophilic substitution to introduce the benzyloxy group at position 7. For example:
- Step 1: Coumarin core formation via Pechmann or Kostanecki-Robinson condensation.
- Step 2: Alkylation using benzyl bromide or substituted benzyl halides under basic conditions (e.g., K₂CO₃ in DMF) to install the benzyloxy group .
- Step 3: Trifluoromethylation at position 4 via electrophilic substitution or cross-coupling reactions (e.g., using CF₃Cu reagents) .
Key Optimization: Reaction temperature (60–80°C) and solvent polarity (DMF or acetone) significantly influence yield. Evidence shows that bromoalkane chain length in analogous compounds affects reactivity, with longer chains (e.g., 1,4-dibromobutane) yielding >90% in some cases .
Q. Basic: Which spectroscopic and crystallographic methods are used to characterize this compound?
- ESI-MS: Confirms molecular weight (e.g., [M+H]⁺ peaks at ~365–440 m/z for derivatives) .
- ¹H/¹³C NMR: Identifies substituent positions:
- X-ray Crystallography: Resolves crystal packing and bond angles (e.g., C–O–C angles ~120° for benzyloxy groups) .
Q. Advanced: How can researchers design assays to evaluate its biological activity, such as enzyme inhibition?
- MAO-B Inhibition Assay:
- Cytotoxicity Screening: Use MTT assays on cancer cell lines (e.g., intrahepatic cholangiocarcinoma). Compare EC₅₀ values with controls like gemcitabine .
Q. Advanced: What strategies resolve contradictions in synthetic yields across similar derivatives?
- Variable Reactivity: Longer bromoalkanes (e.g., 1,4-dibromobutane) improve yields (91%) compared to shorter chains (83–85%) due to reduced steric hindrance .
- Catalyst Optimization: FeCl₃ in THF enhances one-pot reactions for coumarin derivatives, but yield discrepancies may arise from substituent electronic effects (e.g., electron-withdrawing groups slow nucleophilic attack) .
Q. Advanced: How do photophysical properties (e.g., charge transfer) influence its application in sensing?
- Intramolecular Charge Transfer (ICT): The benzyloxy and trifluoromethyl groups create a polarized π-system, enabling fluorescence quenching upon metal binding (e.g., Cr(VI)).
- Applications:
- Cr(VI) Detection: Complexation with Cr(VI) in aqueous solutions shifts absorption maxima (λmax ~450 nm), with a linear range of 0.1–10 µM .
- ROS Probes: Derivatives like 7-hydroxy-4-(trifluoromethyl)-2H-chromen-2-one exhibit fluorescence activation (λex/λem = 340/500 nm) upon ROS interaction .
Q. Advanced: What computational methods support structure-activity relationship (SAR) studies?
- Docking Simulations: Analyze binding interactions with MAO-B (PDB: 2V5Z). The trifluoromethyl group occupies a hydrophobic pocket, while the benzyloxy moiety stabilizes via π-π stacking with Tyr398 .
- DFT Calculations: Predict charge distribution and HOMO-LUMO gaps to optimize electron-withdrawing/-donating substituents for enhanced bioactivity .
Q. Advanced: How is this compound utilized in cellular studies, such as ROS detection?
- Probe Design: Synthesize boronate-protected derivatives (e.g., 7-(4,4,5,5-tetramethyl-1,3,2-dioxaborolan-2-yl)-4-(trifluoromethyl)-2H-chromen-2-one).
- Protocol: Incubate with human mesenchymal stem cells. Intracellular ROS cleave the boronate group, releasing fluorescent 7-hydroxy-4-(trifluoromethyl)-2H-chromen-2-one, detectable via confocal microscopy .
Q. Basic: What are the stability considerations for storage and handling?
- Storage: -20°C under inert atmosphere (N₂/Ar) to prevent hydrolysis of the benzyloxy group.
- Degradation Pathways: Exposure to moisture or light may cause cleavage of the ether bond or oxidation of the coumarin core. Monitor via TLC or HPLC .
Q. Advanced: How does substituent variation at position 3 impact biological activity?
- Case Study: Introducing a 3-(4-trifluoromethylbenzyl) group enhances anti-cancer activity (EC₅₀ = 2.1 µM vs. 8.2 µM for unsubstituted analogs) by improving membrane permeability .
- SAR Trend: Bulky substituents at position 3 reduce MAO-B affinity due to steric clashes, while small polar groups (e.g., -NH₂) improve selectivity .
Q. Advanced: What analytical challenges arise in quantifying this compound in environmental samples?
- Matrix Effects: Soil/water samples require SPE (solid-phase extraction) to remove interfering humic acids.
- Detection Limits: Achieve 0.1 ppb sensitivity via HPLC-UV/Vis with a C18 column (mobile phase: MeCN/H₂O, 70:30 v/v) .
Retrosynthesis Analysis
AI-Powered Synthesis Planning: Our tool employs the Template_relevance Pistachio, Template_relevance Bkms_metabolic, Template_relevance Pistachio_ringbreaker, Template_relevance Reaxys, Template_relevance Reaxys_biocatalysis model, leveraging a vast database of chemical reactions to predict feasible synthetic routes.
One-Step Synthesis Focus: Specifically designed for one-step synthesis, it provides concise and direct routes for your target compounds, streamlining the synthesis process.
Accurate Predictions: Utilizing the extensive PISTACHIO, BKMS_METABOLIC, PISTACHIO_RINGBREAKER, REAXYS, REAXYS_BIOCATALYSIS database, our tool offers high-accuracy predictions, reflecting the latest in chemical research and data.
Strategy Settings
Precursor scoring | Relevance Heuristic |
---|---|
Min. plausibility | 0.01 |
Model | Template_relevance |
Template Set | Pistachio/Bkms_metabolic/Pistachio_ringbreaker/Reaxys/Reaxys_biocatalysis |
Top-N result to add to graph | 6 |
Feasible Synthetic Routes
Featured Recommendations
Most viewed | ||
---|---|---|
Most popular with customers |
Disclaimer and Information on In-Vitro Research Products
Please be aware that all articles and product information presented on BenchChem are intended solely for informational purposes. The products available for purchase on BenchChem are specifically designed for in-vitro studies, which are conducted outside of living organisms. In-vitro studies, derived from the Latin term "in glass," involve experiments performed in controlled laboratory settings using cells or tissues. It is important to note that these products are not categorized as medicines or drugs, and they have not received approval from the FDA for the prevention, treatment, or cure of any medical condition, ailment, or disease. We must emphasize that any form of bodily introduction of these products into humans or animals is strictly prohibited by law. It is essential to adhere to these guidelines to ensure compliance with legal and ethical standards in research and experimentation.