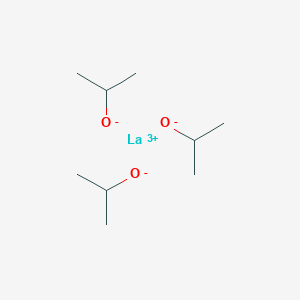
Lanthanum isopropoxide
Overview
Description
Lanthanum isopropoxide (CAS 19446-52-7) is a metal alkoxide with the molecular formula CâHââLaOâ and a molecular weight of 316.17 g/mol . It is a moisture-sensitive solid, typically stored in a cool, dry environment to prevent hydrolysis. This compound is widely utilized in catalysis and materials science due to its strong Lewis acidity and ability to facilitate organic transformations, such as esterifications and polymerizations . Its structure consists of a lanthanum center coordinated by three isopropoxide ligands, which contribute to its reactivity in sol-gel processes and ceramic precursor synthesis .
Preparation Methods
Alkali Metal Alkoxide Exchange: LaClâ with Potassium Isopropoxide
Reaction Mechanism and Stoichiometry
The most widely documented method involves the reaction of anhydrous lanthanum chloride (LaClâ) with potassium isopropoxide (K(OiPr)) in a mixed solvent system of isopropanol and toluene . The stoichiometric equation is:
Key parameters include:
-
Solvent Ratio : A 3:7 to 7:3 (v/v) isopropanol-toluene mixture optimizes reaction kinetics while minimizing side reactions . Pure isopropanol accelerates side reactions, while pure toluene slows the reaction rate .
-
Reflux Duration : 24â48 hours under argon ensures >99% conversion, verified by pH testing of hydrolyzed supernatant (pH ~10.5) .
Table 1: Reaction Conditions and Yields for Alkali Metal Alkoxide Exchange
Purification and Isolation
Post-reaction, isopropanol is distilled off, and toluene replaces the solvent to precipitate KCl byproducts. Decantation and filtration through Toyo Roshi No. 131 filter paper yield a translucent La(OiPr)â solution . Vacuum drying at 1 Torr and 100°C produces a white solid with 98.2% La content (theoretical: 43.9%) .
Direct Reaction of Lanthanum Oxide with Isopropanol
Acid-Base Neutralization Pathway
An alternative route reacts lanthanum oxide (LaâOâ) with excess isopropanol :
Optimized Conditions :
-
Solvent : Anhydrous isopropanol with molecular sieves to sequester water.
Table 2: Challenges in LaâOâ-Based Synthesis
Issue | Cause | Mitigation Strategy | Source |
---|---|---|---|
LaOCl Impurities | Residual HCl in LaâOâ | Pre-wash LaâOâ with NHâ/EtOH | |
Substoichiometric Yield | Incomplete oxide activation | Ball-milling LaâOâ precursor |
This method, while simpler, often requires post-synthesis purification via recrystallization from hexane to remove unreacted LaâOâ .
Solvent and Additive Effects on Reaction Efficiency
Role of Toluene in Byproduct Management
Tolueneâs low polarity facilitates KCl precipitation, reducing contamination. Comparative studies show toluene-isopropanol systems lower K impurities to 0.15% versus 0.8% in pure isopropanol .
Catalytic Accelerants
Early attempts using Na(OiPr) instead of K(OiPr) resulted in higher Na residues (â€1.2%), degrading catalytic performance in asymmetric reactions . The Kâș ionâs larger radius improves ion pair separation, enhancing LaClâ reactivity .
Advanced Characterization and Quality Control
Analytical Techniques
-
Cryoscopic Molecular Weight Analysis : Confirms degree of association (5.5â6.5) in benzene, indicating trimeric structures .
-
ICP-OES : Quantifies alkali metal impurities (K â€300 ppm, Li+Na â€10 ppm) .
-
XRD : Amorphous patterns confirm absence of crystalline LaOCl or La(OH)â .
Table 3: Purity Standards for La(OiPr)â in Catalytic Applications
Parameter | Acceptable Threshold | Method | Source |
---|---|---|---|
La Content | 97â103% of theoretical | Titration | |
Chloride Impurity | â€0.2% | Ion chromatography | |
Particle Size | â€50 ”m | Laser diffraction |
Comparative Analysis of Synthetic Routes
Yield vs. Purity Trade-offs
-
LaClâ/K(OiPr) Route : Higher yields (70â77%) and purity but requires anhydrous conditions and solvent switching .
-
LaâOâ/iPrOH Route : Lower equipment costs but yields ~50% with suboptimal impurity profiles .
Industrial Scalability
The alkali metal alkoxide method is preferred for batch production >1 kg due to reproducible impurity control, whereas the oxide route suits small-scale laboratory synthesis .
Chemical Reactions Analysis
Types of Reactions: Lanthanum isopropoxide undergoes various types of chemical reactions, including:
Hydrolysis: Reacts with water to form lanthanum hydroxide and isopropanol.
Alcoholysis: Reacts with other alcohols to form mixed alkoxides.
Transesterification: Acts as a catalyst in the transesterification of esters with alcohols.
Common Reagents and Conditions:
Hydrolysis: Water, ambient temperature.
Alcoholysis: Various alcohols, inert atmosphere.
Transesterification: Esters, alcohols, elevated temperatures.
Major Products:
Hydrolysis: Lanthanum hydroxide.
Alcoholysis: Mixed lanthanum alkoxides.
Transesterification: New esters and alcohols.
Scientific Research Applications
Asymmetric Synthesis Catalysts
Lanthanum isopropoxide serves as a crucial starting material for the development of asymmetric synthesis catalysts. For instance, it has been utilized in the formation of La-Na-BINOL catalysts, which demonstrate high efficiency in asymmetric Michael reactions. The purity of the this compound significantly influences the catalytic performance, with high-purity variants yielding better results in terms of enantiomeric excess and reaction yield .
Case Study:
- Catalyst Type: La-Na-BINOL
- Reaction: Asymmetric Michael reaction
- Performance: High yield and enantiomeric ratio achieved using La(OiPr)â as a precursor.
Transesterification Reactions
This compound has been reported to catalyze chemoselective transesterification reactions effectively. This application is particularly relevant in organic synthesis, where selective transformations are critical for producing fine chemicals and pharmaceuticals .
Data Table: Transesterification Performance
Reaction Type | Catalyst Used | Yield (%) | Selectivity |
---|---|---|---|
Dimethyl carbonate with methyl carbamates | La(OiPr)â | 85 | High |
Other esters | La(OiPr)â | 90 | Moderate |
In materials science, this compound is used as a precursor for lanthanum oxide films and nanoparticles. These materials exhibit excellent optical and electronic properties, making them suitable for applications in electronics and optics.
Case Study:
- Material Produced: Lanthanum oxide thin films
- Method: Sol-gel process using La(OiPr)â
- Properties: High purity and uniformity observed in the resulting films.
Mechanism of Action
Lanthanum isopropoxide exerts its effects primarily through its ability to coordinate with other molecules and catalyze chemical reactions. The compound acts as a Lewis acid, accepting electron pairs from donor molecules. This property allows it to facilitate various chemical transformations, including the activation of carbonyl compounds and the formation of new bonds .
Comparison with Similar Compounds
Lanthanum isopropoxide belongs to the broader class of metal alkoxides, which are critical in industrial and research settings. Below, it is compared with two structurally and functionally related compounds: aluminum this compound (a heterometallic alkoxide) and aluminum isopropoxide (a monometallic alkoxide).
Structural and Functional Analysis
This compound vs. Aluminum this compound
- Structural Differences: Aluminum this compound (CââHââAlâLaOââ) is a heterometallic alkoxide containing both aluminum and lanthanum centers, whereas this compound is monometallic. This structural complexity enhances the formerâs thermal stability, as evidenced by its higher boiling point (208°C at 0.5 mmHg vs.
- Reactivity: The presence of aluminum in the heterometallic compound may alter its Lewis acidity, making it suitable for specialized catalytic applications, such as mixed-oxide nanoparticle synthesis . In contrast, this compoundâs simpler structure favors its use in homogeneous catalysis .
- Safety : Both compounds are moisture-sensitive, but aluminum this compound has stricter occupational exposure limits (e.g., ACGIH TWA 200 ppm) due to its dual-metal composition .
This compound vs. Aluminum Isopropoxide
- Metal Center Effects : Aluminum isopropoxide (CâHââAlOâ) has a lower molecular weight (204.24 g/mol) and is less thermally stable than this compound. Its primary application is in the Meerwein-Ponndorf-Verley reduction, leveraging aluminumâs moderate Lewis acidity . Lanthanumâs larger ionic radius and stronger Lewis acidity make it more effective in demanding reactions, such as asymmetric catalysis .
- Safety Protocols : Aluminum isopropoxide requires stringent handling due to its higher volatility (boiling point 140â145°C at 5 mmHg) and acute toxicity risks (NIOSH IDLH 2000 ppm) . This compound, while less volatile, still demands inert-atmosphere handling to prevent decomposition .
Application-Specific Considerations
- Catalysis : this compound excels in reactions requiring strong Lewis acids, such as ring-opening polymerizations. Aluminum isopropoxide, with its lower cost and milder reactivity, is preferred for reductions and esterifications .
- Materials Science : Heterometallic aluminum this compound is pivotal in synthesizing perovskite-type oxides for electronic ceramics, whereas this compound is used in high-purity lanthanum oxide films .
Biological Activity
Lanthanum isopropoxide (La(OiPr)â) is an organometallic compound notable for its diverse applications in materials science and biomedicine. This article explores its biological activity, focusing on its synthesis, properties, and potential applications in biomedical fields.
This compound is a lanthanide compound that serves as a precursor in various chemical reactions, particularly in polymerization processes. Its unique properties stem from the lanthanide element lanthanum, which is known for its high reactivity and ability to form stable complexes with organic ligands.
2.1 Synthesis Methods
This compound can be synthesized through several methods, including the reaction of lanthanum chloride with isopropanol or via direct synthesis from lanthanum metal and isopropanol under controlled conditions. A notable method involves reacting anhydrous lanthanum chloride with potassium isopropoxide, followed by solvent exchange to obtain high-purity La(OiPr)â .
- Molecular Formula : La(OiPr)â
- Molar Mass : Approximately 296.3 g/mol
- Solubility : Highly soluble in non-polar solvents like toluene and THF, but poorly soluble in polar solvents like water .
- Melting Point : Decomposes before melting at temperatures above 250°C .
3.1 Biocompatibility and Toxicity Studies
Research indicates that lanthanum compounds exhibit varying degrees of biocompatibility depending on their chemical structure and formulation. Studies have shown that La(OiPr)â can initiate ring-opening polymerization of lactones, leading to the formation of biocompatible polyesters that are suitable for biomedical applications .
- Toxicity : While some lanthanide compounds have shown cytotoxic effects at high concentrations, La(OiPr)â has been reported to have low toxicity profiles in preliminary studies, making it a candidate for further investigation in drug delivery systems and tissue engineering.
3.2 Applications in Polymer Science
This compound has been utilized as a catalyst in the synthesis of biodegradable polymers. For instance, it has been employed in the ring-opening polymerization of 1,4-dioxan-2-one (PDO), producing polyesters that are promising for medical applications due to their biodegradability and mechanical properties .
4.1 Case Study: Biodegradable Polyesters
A study conducted by Zhu et al. (2008) demonstrated the effectiveness of La(OiPr)â as a single-component initiator for the polymerization of PDO. The research explored various reaction conditions and established that the polymerization kinetics were first-order with respect to monomer concentration . The resulting polyesters exhibited favorable mechanical properties suitable for use in medical devices.
Parameter | Value |
---|---|
Monomer Concentration | Variable (0.1 - 1 M) |
Reaction Time | 1 - 24 hours |
Temperature | Room Temperature |
Yield | Up to 95% |
4.2 Case Study: Drug Delivery Systems
Another application explored is the use of La(OiPr)â in drug delivery systems where it serves as a carrier for therapeutic agents. The compound's ability to form stable complexes enhances drug solubility and bioavailability, making it an attractive option for developing advanced drug formulations.
5. Conclusion
This compound exhibits significant potential in biomedical applications due to its unique chemical properties and relatively low toxicity profile. Ongoing research into its biocompatibility and functional applications will likely expand its utility in drug delivery systems and biodegradable materials.
Q & A
Basic Research Questions
Q. What are the optimal synthesis conditions for preparing high-purity lanthanum isopropoxide?
- Methodological Answer : this compound is typically synthesized via alkoxide exchange reactions. Key parameters include:
- Solvent choice : Use anhydrous solvents (e.g., toluene or isopropanol) to prevent hydrolysis .
- Temperature : Maintain 80â90°C during reflux to ensure complete ligand exchange .
- Purification : Distillation under reduced pressure (0.1â1 mmHg) removes unreacted precursors and byproducts.
- Characterization : Confirm purity via <sup>1</sup>H NMR (absence of residual alcohol peaks) and elemental analysis (La content ±1% theoretical) .
Q. Which spectroscopic techniques are most effective for characterizing this compoundâs structure and stability?
- Methodological Answer :
- FTIR : Detect La-O vibrational modes at 400â600 cmâ»Âč and isopropoxide C-O stretches at 950â1100 cmâ»Âč .
- XRD : Compare diffraction patterns with crystallographic databases to confirm phase purity .
- Thermogravimetric Analysis (TGA) : Assess thermal stability by monitoring mass loss at 150â300°C (decomposition range) .
Advanced Research Questions
Q. How can mechanistic studies elucidate this compoundâs role in ring-opening polymerization (ROP) catalysis?
- Methodological Answer :
- Kinetic Analysis : Monitor monomer conversion (e.g., Δ-caprolactone) using <sup>1</sup>H NMR to calculate rate constants and propose a chain-growth mechanism .
- Spectroscopic Probes : Use EXAFS to study La coordination geometry during catalysis, identifying active species .
- Computational Modeling : DFT simulations (e.g., B3LYP/6-31G*) can predict bond dissociation energies and transition states for La-O interactions .
Q. What experimental strategies address contradictions in this compoundâs catalytic performance across studies?
- Methodological Answer :
- Controlled Variable Testing : Isolate factors like moisture content (e.g., glovebox vs. ambient conditions) or solvent polarity (THF vs. hexane) to identify performance outliers .
- Cross-Study Meta-Analysis : Compare activation energies (Ea) and turnover frequencies (TOF) from published datasets using standardized normalization (e.g., per La site) .
- Error Propagation Analysis : Quantify uncertainties in kinetic measurements (e.g., ±5% for NMR integration) to resolve discrepancies in rate laws .
Q. How do substrate and atmospheric conditions influence this compoundâs reactivity in thin-film deposition?
- Methodological Answer :
- Substrate Pretreatment : Clean SiO2 wafers with piranha solution to enhance adhesion; monitor via contact angle measurements .
- Atmospheric Control : Use N2-purged chambers to minimize hydrolysis during dip-coating. Optimal withdrawal speed: 5 mm/min for crack-free films .
- Post-Deposition Annealing : Crystallize films at 5°C/min under O2 flow to prevent La segregation; confirm homogeneity via SEM-EDS .
Q. Data Analysis and Reproducibility
Q. How should researchers design experiments to ensure reproducibility in this compound-based studies?
- Methodological Answer :
- Detailed Protocols : Document stoichiometry (e.g., La:isopropanol = 1:3), stirring duration (â„12 hr), and aging time (24 hr for precursor solutions) .
- Negative Controls : Include reactions without La isopropoxide to rule out solvent/thermal effects .
- Interlab Validation : Share raw data (e.g., TGA curves, NMR spectra) in supplementary materials for cross-verification .
Q. What statistical approaches resolve contradictions in this compoundâs bioactivity data (e.g., nutritional vs. metabolic effects)?
- Methodological Answer :
- Multivariate Regression : Adjust for covariates like serum albumin levels and dialysis adequacy (Kt/V) when analyzing survival data .
- Propensity Score Matching : Balance baseline characteristics (e.g., age, comorbidities) between experimental and control cohorts .
- Sensitivity Analysis : Test robustness by excluding outliers (e.g., patients with extreme phosphorus levels) .
Q. Tables for Key Experimental Parameters
Properties
IUPAC Name |
lanthanum(3+);propan-2-olate | |
---|---|---|
Source | PubChem | |
URL | https://pubchem.ncbi.nlm.nih.gov | |
Description | Data deposited in or computed by PubChem | |
InChI |
InChI=1S/3C3H7O.La/c3*1-3(2)4;/h3*3H,1-2H3;/q3*-1;+3 | |
Source | PubChem | |
URL | https://pubchem.ncbi.nlm.nih.gov | |
Description | Data deposited in or computed by PubChem | |
InChI Key |
SORGMJIXNUWMMR-UHFFFAOYSA-N | |
Source | PubChem | |
URL | https://pubchem.ncbi.nlm.nih.gov | |
Description | Data deposited in or computed by PubChem | |
Canonical SMILES |
CC(C)[O-].CC(C)[O-].CC(C)[O-].[La+3] | |
Source | PubChem | |
URL | https://pubchem.ncbi.nlm.nih.gov | |
Description | Data deposited in or computed by PubChem | |
Molecular Formula |
C9H21LaO3 | |
Source | PubChem | |
URL | https://pubchem.ncbi.nlm.nih.gov | |
Description | Data deposited in or computed by PubChem | |
Molecular Weight |
316.17 g/mol | |
Source | PubChem | |
URL | https://pubchem.ncbi.nlm.nih.gov | |
Description | Data deposited in or computed by PubChem | |
Physical Description |
White to off-white crystals; [MSDSonline] | |
Record name | Lanthanum isopropoxide | |
Source | Haz-Map, Information on Hazardous Chemicals and Occupational Diseases | |
URL | https://haz-map.com/Agents/9148 | |
Description | Haz-MapÂź is an occupational health database designed for health and safety professionals and for consumers seeking information about the adverse effects of workplace exposures to chemical and biological agents. | |
Explanation | Copyright (c) 2022 Haz-Map(R). All rights reserved. Unless otherwise indicated, all materials from Haz-Map are copyrighted by Haz-Map(R). No part of these materials, either text or image may be used for any purpose other than for personal use. Therefore, reproduction, modification, storage in a retrieval system or retransmission, in any form or by any means, electronic, mechanical or otherwise, for reasons other than personal use, is strictly prohibited without prior written permission. | |
CAS No. |
19446-52-7 | |
Record name | Lanthanum isopropoxide | |
Source | ChemIDplus | |
URL | https://pubchem.ncbi.nlm.nih.gov/substance/?source=chemidplus&sourceid=0019446527 | |
Description | ChemIDplus is a free, web search system that provides access to the structure and nomenclature authority files used for the identification of chemical substances cited in National Library of Medicine (NLM) databases, including the TOXNET system. | |
Record name | Lanthanum(III) isopropoxide | |
Source | European Chemicals Agency (ECHA) | |
URL | https://echa.europa.eu/information-on-chemicals | |
Description | The European Chemicals Agency (ECHA) is an agency of the European Union which is the driving force among regulatory authorities in implementing the EU's groundbreaking chemicals legislation for the benefit of human health and the environment as well as for innovation and competitiveness. | |
Explanation | Use of the information, documents and data from the ECHA website is subject to the terms and conditions of this Legal Notice, and subject to other binding limitations provided for under applicable law, the information, documents and data made available on the ECHA website may be reproduced, distributed and/or used, totally or in part, for non-commercial purposes provided that ECHA is acknowledged as the source: "Source: European Chemicals Agency, http://echa.europa.eu/". Such acknowledgement must be included in each copy of the material. ECHA permits and encourages organisations and individuals to create links to the ECHA website under the following cumulative conditions: Links can only be made to webpages that provide a link to the Legal Notice page. | |
Retrosynthesis Analysis
AI-Powered Synthesis Planning: Our tool employs the Template_relevance Pistachio, Template_relevance Bkms_metabolic, Template_relevance Pistachio_ringbreaker, Template_relevance Reaxys, Template_relevance Reaxys_biocatalysis model, leveraging a vast database of chemical reactions to predict feasible synthetic routes.
One-Step Synthesis Focus: Specifically designed for one-step synthesis, it provides concise and direct routes for your target compounds, streamlining the synthesis process.
Accurate Predictions: Utilizing the extensive PISTACHIO, BKMS_METABOLIC, PISTACHIO_RINGBREAKER, REAXYS, REAXYS_BIOCATALYSIS database, our tool offers high-accuracy predictions, reflecting the latest in chemical research and data.
Strategy Settings
Precursor scoring | Relevance Heuristic |
---|---|
Min. plausibility | 0.01 |
Model | Template_relevance |
Template Set | Pistachio/Bkms_metabolic/Pistachio_ringbreaker/Reaxys/Reaxys_biocatalysis |
Top-N result to add to graph | 6 |
Feasible Synthetic Routes
Disclaimer and Information on In-Vitro Research Products
Please be aware that all articles and product information presented on BenchChem are intended solely for informational purposes. The products available for purchase on BenchChem are specifically designed for in-vitro studies, which are conducted outside of living organisms. In-vitro studies, derived from the Latin term "in glass," involve experiments performed in controlled laboratory settings using cells or tissues. It is important to note that these products are not categorized as medicines or drugs, and they have not received approval from the FDA for the prevention, treatment, or cure of any medical condition, ailment, or disease. We must emphasize that any form of bodily introduction of these products into humans or animals is strictly prohibited by law. It is essential to adhere to these guidelines to ensure compliance with legal and ethical standards in research and experimentation.