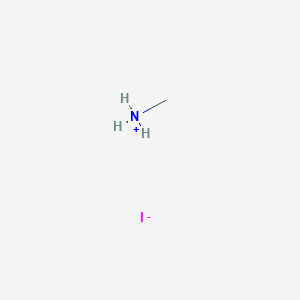
Methylammonium iodide
Descripción general
Descripción
Methylammonium iodide is an organic halide with a formula of CH3NH3I. It is an ammonium salt composed of methylamine and hydrogen iodide . The primary application for methylammonium iodide, sometimes in combination with other methylammonium halides, is as a component of perovskite crystalline solar cells .
Synthesis Analysis
Methylammonium iodide (MAI) is a precursor for the synthesis of organic-inorganic hybrid perovskites . A modified solution deposition method has been used to synthesize uniform methylammonium lead iodide (MAPbI3) nanoplates, nanocubes, and nanorods . The morphology can be controlled by regulating the amounts of reactant MAI and the rate at which MAPbI3 precursor is added into toluene solution .Molecular Structure Analysis
The monomer and dimer structures of methylammonium iodide have been investigated theoretically . The calculations show that the hydrogen bonds are found to be the major factor that stabilizes the methylammonium iodide monomers and dimers . The dimeric structure exhibits geometry with each iodine atom shared by two neighboring hydrogen atoms in the methylammonium cation molecules .Chemical Reactions Analysis
The hydrogen bonds in the methylammonium iodide are proposed to be correlated with the three-dimensional halide perovskite buildup . The formation of the hydrogen bond that is available in the halide perovskite framework could be “historically” traced back as early as the precursor preparation .Physical And Chemical Properties Analysis
Methylammonium iodide has a molar mass of 158.96951 g/mol and appears as a white powder . It exhibits a broad absorption range and a band-gap of 1.6 eV .Aplicaciones Científicas De Investigación
Specific Scientific Field
This application falls under the field of Renewable Energy , specifically Photovoltaics .
Summary of the Application
Methylammonium iodide is a precursor for the synthesis of halide perovskite materials, which have received much attention in recent years for their applications in solar cells . These materials are used in perovskite solar cells, which have shown remarkable progress in power conversion efficiencies .
Methods of Application or Experimental Procedures
The synthesis of halide perovskite materials involves the use of methylammonium iodide as a precursor. The precursor is used in the formation of the halide perovskite structure, with hydrogen bonds playing a major role in stabilizing the structure . In a specific study, the equimolar precursor ratio of the organic halide (methylammonium iodide; MAI) and metal halide (lead iodide; PbI2) was tuned in a fixed solvent mixture of N,N -dimethylformamide (DMF):dimethylsulfoxide (DMSO) .
Results or Outcomes
The use of methylammonium iodide in the synthesis of halide perovskite materials has led to significant improvements in the efficiency of solar cells. For instance, perovskite solar cells based on methylammonium lead iodide (MALI) have achieved efficiencies as high as 26% . In the specific study mentioned above, the perovskite solar cells fabricated from the (1.5:1.5) formulation exhibited a power conversion efficiency (PCE) of 14.56% .
Application in Light-Emitting Diodes (LEDs)
Specific Scientific Field
This application falls under the field of Optoelectronics .
Summary of the Application
Methylammonium iodide is used in the synthesis of halide perovskite materials, which have applications in light-emitting diodes (LEDs) .
Methods of Application or Experimental Procedures
The synthesis of these materials involves the use of methylammonium iodide as a precursor. The precursor is used in the formation of the halide perovskite structure .
Results or Outcomes
The use of methylammonium iodide in the synthesis of halide perovskite materials has led to advancements in the field of optoelectronics, particularly in the development of LEDs .
Application in Quantum Chemistry
Specific Scientific Field
This application falls under the field of Quantum Chemistry .
Summary of the Application
Methylammonium iodide is used as a precursor for the synthesis of halide perovskite materials. These materials have been studied extensively in quantum chemistry to understand their structures and impacts on halide perovskite properties .
Methods of Application or Experimental Procedures
Theoretical investigations have been conducted on the monomer and dimer structures of methylammonium iodide, a precursor for the synthesis of the halide perovskite CH3NH3PbI3 . The calculations show that hydrogen bonds are the major factor that stabilizes the methylammonium iodide monomers and dimers .
Results or Outcomes
The study provides a more complete view of the halide perovskite structures and addresses the importance of the hydrogen bonds in the synthesis and the crystal formation of halide perovskite materials .
Application in Opto-Electronic Devices
Specific Scientific Field
This application falls under the field of Opto-Electronics .
Summary of the Application
Methylammonium iodide is used in the synthesis of halide perovskite materials, which have applications in opto-electronic devices .
Methods of Application or Experimental Procedures
The synthesis of these materials involves the use of methylammonium iodide as a precursor. The precursor is used in the formation of the halide perovskite structure .
Results or Outcomes
The use of methylammonium iodide in the synthesis of halide perovskite materials has led to advancements in the field of opto-electronics, particularly in the development of opto-electronic devices .
Application in Quantum Mechanics
Specific Scientific Field
This application falls under the field of Quantum Mechanics .
Summary of the Application
Methylammonium iodide is used as a precursor for the synthesis of halide perovskite materials. These materials have been studied extensively in quantum mechanics to understand their structures and impacts on halide perovskite properties .
Methods of Application or Experimental Procedures
Theoretical investigations have been conducted on the monomer and dimer structures of methylammonium iodide, a precursor for the synthesis of the halide perovskite CH3NH3PbI3 . The calculations show that hydrogen bonds are the major factor that stabilizes the methylammonium iodide monomers and dimers .
Results or Outcomes
The study provides a more complete view of the halide perovskite structures and addresses the importance of the hydrogen bonds in the synthesis and the crystal formation of halide perovskite materials .
Application in Photovoltaic Devices
Specific Scientific Field
This application falls under the field of Photovoltaics .
Summary of the Application
Methylammonium iodide is used in the synthesis of halide perovskite materials, which have applications in photovoltaic devices .
Methods of Application or Experimental Procedures
The synthesis of these materials involves the use of methylammonium iodide as a precursor. The precursor is used in the formation of the halide perovskite structure .
Results or Outcomes
The use of methylammonium iodide in the synthesis of halide perovskite materials has led to advancements in the field of photovoltaics, particularly in the development of photovoltaic devices .
Safety And Hazards
Direcciones Futuras
Propiedades
IUPAC Name |
methylazanium;iodide | |
---|---|---|
Source | PubChem | |
URL | https://pubchem.ncbi.nlm.nih.gov | |
Description | Data deposited in or computed by PubChem | |
InChI |
InChI=1S/CH5N.HI/c1-2;/h2H2,1H3;1H | |
Source | PubChem | |
URL | https://pubchem.ncbi.nlm.nih.gov | |
Description | Data deposited in or computed by PubChem | |
InChI Key |
LLWRXQXPJMPHLR-UHFFFAOYSA-N | |
Source | PubChem | |
URL | https://pubchem.ncbi.nlm.nih.gov | |
Description | Data deposited in or computed by PubChem | |
Canonical SMILES |
C[NH3+].[I-] | |
Source | PubChem | |
URL | https://pubchem.ncbi.nlm.nih.gov | |
Description | Data deposited in or computed by PubChem | |
Molecular Formula |
CH6IN | |
Source | PubChem | |
URL | https://pubchem.ncbi.nlm.nih.gov | |
Description | Data deposited in or computed by PubChem | |
Related CAS |
74-89-5 (Parent) | |
Record name | Methylammonium iodide | |
Source | ChemIDplus | |
URL | https://pubchem.ncbi.nlm.nih.gov/substance/?source=chemidplus&sourceid=0014965492 | |
Description | ChemIDplus is a free, web search system that provides access to the structure and nomenclature authority files used for the identification of chemical substances cited in National Library of Medicine (NLM) databases, including the TOXNET system. | |
Molecular Weight |
158.970 g/mol | |
Source | PubChem | |
URL | https://pubchem.ncbi.nlm.nih.gov | |
Description | Data deposited in or computed by PubChem | |
Product Name |
Methylammonium iodide | |
CAS RN |
14965-49-2 | |
Record name | Methanamine, hydriodide | |
Source | CAS Common Chemistry | |
URL | https://commonchemistry.cas.org/detail?cas_rn=14965-49-2 | |
Description | CAS Common Chemistry is an open community resource for accessing chemical information. Nearly 500,000 chemical substances from CAS REGISTRY cover areas of community interest, including common and frequently regulated chemicals, and those relevant to high school and undergraduate chemistry classes. This chemical information, curated by our expert scientists, is provided in alignment with our mission as a division of the American Chemical Society. | |
Explanation | The data from CAS Common Chemistry is provided under a CC-BY-NC 4.0 license, unless otherwise stated. | |
Record name | Methylammonium iodide | |
Source | ChemIDplus | |
URL | https://pubchem.ncbi.nlm.nih.gov/substance/?source=chemidplus&sourceid=0014965492 | |
Description | ChemIDplus is a free, web search system that provides access to the structure and nomenclature authority files used for the identification of chemical substances cited in National Library of Medicine (NLM) databases, including the TOXNET system. | |
Record name | Methylammonium iodide | |
Source | European Chemicals Agency (ECHA) | |
URL | https://echa.europa.eu/substance-information/-/substanceinfo/100.035.474 | |
Description | The European Chemicals Agency (ECHA) is an agency of the European Union which is the driving force among regulatory authorities in implementing the EU's groundbreaking chemicals legislation for the benefit of human health and the environment as well as for innovation and competitiveness. | |
Explanation | Use of the information, documents and data from the ECHA website is subject to the terms and conditions of this Legal Notice, and subject to other binding limitations provided for under applicable law, the information, documents and data made available on the ECHA website may be reproduced, distributed and/or used, totally or in part, for non-commercial purposes provided that ECHA is acknowledged as the source: "Source: European Chemicals Agency, http://echa.europa.eu/". Such acknowledgement must be included in each copy of the material. ECHA permits and encourages organisations and individuals to create links to the ECHA website under the following cumulative conditions: Links can only be made to webpages that provide a link to the Legal Notice page. | |
Retrosynthesis Analysis
AI-Powered Synthesis Planning: Our tool employs the Template_relevance Pistachio, Template_relevance Bkms_metabolic, Template_relevance Pistachio_ringbreaker, Template_relevance Reaxys, Template_relevance Reaxys_biocatalysis model, leveraging a vast database of chemical reactions to predict feasible synthetic routes.
One-Step Synthesis Focus: Specifically designed for one-step synthesis, it provides concise and direct routes for your target compounds, streamlining the synthesis process.
Accurate Predictions: Utilizing the extensive PISTACHIO, BKMS_METABOLIC, PISTACHIO_RINGBREAKER, REAXYS, REAXYS_BIOCATALYSIS database, our tool offers high-accuracy predictions, reflecting the latest in chemical research and data.
Strategy Settings
Precursor scoring | Relevance Heuristic |
---|---|
Min. plausibility | 0.01 |
Model | Template_relevance |
Template Set | Pistachio/Bkms_metabolic/Pistachio_ringbreaker/Reaxys/Reaxys_biocatalysis |
Top-N result to add to graph | 6 |
Feasible Synthetic Routes
Citations
Descargo de responsabilidad e información sobre productos de investigación in vitro
Tenga en cuenta que todos los artículos e información de productos presentados en BenchChem están destinados únicamente con fines informativos. Los productos disponibles para la compra en BenchChem están diseñados específicamente para estudios in vitro, que se realizan fuera de organismos vivos. Los estudios in vitro, derivados del término latino "in vidrio", involucran experimentos realizados en entornos de laboratorio controlados utilizando células o tejidos. Es importante tener en cuenta que estos productos no se clasifican como medicamentos y no han recibido la aprobación de la FDA para la prevención, tratamiento o cura de ninguna condición médica, dolencia o enfermedad. Debemos enfatizar que cualquier forma de introducción corporal de estos productos en humanos o animales está estrictamente prohibida por ley. Es esencial adherirse a estas pautas para garantizar el cumplimiento de los estándares legales y éticos en la investigación y experimentación.