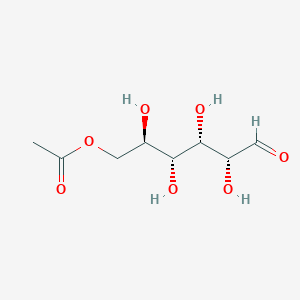
6-O-acetyl-D-glucose
Overview
Description
6-O-Acetyl-D-glucose is a monosaccharide derivative where an acetyl group (-OAc) is attached to the hydroxyl group at the C6 position of D-glucose.
Preparation Methods
Partial Acetylation with Diluted Acetic Acid
The earliest and most straightforward method for synthesizing 6-O-acetyl-D-glucose involves direct esterification of D-glucose with diluted acetic acid. Bílik et al. pioneered this approach, exploiting the higher reactivity of the primary hydroxyl group at the C6 position under mild acidic conditions .
Reaction Conditions and Workflow
A solution of 400 g D-glucose in 75% acetic acid (1,000 mL) is heated at 100°C for 8 hours. The reaction mixture is concentrated under reduced pressure to remove excess acetic acid, followed by crystallization from methanol and ethanol to recover unreacted D-glucose (55–65% yield). Residual glucose is metabolized via yeast fermentation, while di- and tri-O-acetyl byproducts are extracted with ethyl acetate. Final crystallization from ethanol yields this compound in 20–25% yield relative to consumed glucose .
Advantages and Limitations
This method avoids chromatographic purification, making it scalable for industrial applications. However, the moderate yield and need for fermentation to eliminate unreacted substrate limit its efficiency. Side products, though minimal, necessitate careful extraction to isolate the target compound .
Chemoenzymatic Synthesis Using Candida rugosa Lipase
A high-yielding, regioselective route employs Candida rugosa lipase (CRL) to deacetylate peracetylated glucose precursors. This method capitalizes on the enzyme’s specificity for primary hydroxyl groups, enabling precise synthesis of this compound .
Enzymatic Deacetylation Protocol
1,2,3,4,6-Penta-O-acetyl-α-D-glucopyranose is treated with CRL in phosphate buffer (pH 7.0) at 37°C. The lipase selectively hydrolyzes the acetyl group at the C6 position, yielding this compound with >95% regioselectivity. Subsequent purification via recrystallization achieves an overall yield of 77% from α-D-glucose .
Molecular Basis of Selectivity
Molecular modeling studies reveal that CRL’s active site accommodates the glucose backbone in a conformation that positions the C6 acetyl group optimally for hydrolysis. Transition-state analogs demonstrate that steric hindrance at secondary positions (C2, C3, C4) further biases the enzyme toward primary deacetylation .
Industrial Applicability
This method’s efficiency, scalability, and minimal byproduct formation make it ideal for large-scale production. The enzymatic step operates under aqueous, mild conditions, reducing energy consumption and environmental impact .
Regioselective Acetylation via Trityl Protection and Acyl Migration
A sophisticated strategy combines trityl group protection and acyl migration to achieve regioselective acetylation at C6. Hatanaka et al. demonstrated this approach using D-glucose derivatives .
Synthetic Pathway
-
Tritylation : D-glucose is treated with trityl chloride to protect the C6 hydroxyl group, forming 6-O-trityl-D-glucose.
-
Acetylation : Remaining hydroxyl groups (C1, C2, C3, C4) are acetylated using acetic anhydride in pyridine.
-
Detritylation : Trifluoroacetic acid removes the trityl group, inducing acyl migration from C4 to C6.
-
Purification : Column chromatography isolates 1,2,3,6-tetra-O-acetyl-β-D-glucopyranose, which is hydrolyzed to yield this compound .
Yield Optimization
Initial yields of 24% are improved to 70% through recycling of byproducts. The acyl migration step is temperature-sensitive, with optimal performance at 65°C .
Critical Analysis
While this method ensures high regioselectivity, its multi-step nature and reliance on toxic reagents (e.g., trifluoroacetic acid) complicate scalability. Nevertheless, it remains valuable for laboratory-scale synthesis requiring high purity .
Comparative Evaluation of Methods
Chemical Reactions Analysis
Types of Reactions
[(2R,3R,4S,5R)-2,3,4,5-tetrahydroxy-6-oxohexyl] acetate undergoes various chemical reactions, including:
Oxidation: The compound can be oxidized to form corresponding carboxylic acids.
Reduction: Reduction reactions can convert the oxo group to a hydroxyl group.
Substitution: The acetate group can be substituted with other functional groups through nucleophilic substitution reactions.
Common Reagents and Conditions
Oxidation: Common oxidizing agents include potassium permanganate (KMnO4) and chromium trioxide (CrO3).
Reduction: Reducing agents such as sodium borohydride (NaBH4) or lithium aluminum hydride (LiAlH4) are used.
Substitution: Nucleophiles like amines or alcohols can be used for substitution reactions.
Major Products
Oxidation: Carboxylic acids
Reduction: Alcohols
Substitution: Various substituted derivatives depending on the nucleophile used
Scientific Research Applications
[(2R,3R,4S,5R)-2,3,4,5-tetrahydroxy-6-oxohexyl] acetate has a wide range of applications in scientific research:
Chemistry: Used as a building block in organic synthesis and as a reagent in various chemical reactions.
Biology: Studied for its role in metabolic pathways and as a potential biomarker.
Medicine: Investigated for its potential therapeutic effects and as a precursor in drug synthesis.
Industry: Utilized in the production of specialty chemicals and as an intermediate in the synthesis of complex molecules.
Mechanism of Action
The mechanism of action of [(2R,3R,4S,5R)-2,3,4,5-tetrahydroxy-6-oxohexyl] acetate involves its interaction with specific molecular targets and pathways. The compound can act as a substrate for various enzymes, leading to the formation of different metabolites. These metabolites can then participate in biochemical pathways, exerting their effects on cellular processes.
Comparison with Similar Compounds
Comparison with Structurally Similar Compounds
Structural and Functional Overview
The table below compares 6-O-acetyl-D-glucose with related monosaccharide derivatives:
Key Differences and Implications
Substituent Position and Type :
- This compound vs. 3-O-Methyl-D-glucose : Acetylation at C6 vs. methylation at C3 leads to divergent biological roles. While this compound affects ion fluxes in pancreatic cells, 3-O-methyl-D-glucose directly inhibits insulin secretion .
- This compound vs. 6-O-Galloylglucose : The galloyl group in the latter increases molecular weight and hydrophobicity, enabling solubility in organic solvents (e.g., chloroform) and pharmacological applications .
Synthetic Accessibility :
- This compound is synthesized in moderate yields (10–30%) via direct acetylation , whereas 6-O-galloylglucose requires multi-step enzymatic or chemical galloylation .
- 6-Chloro-6-deoxy-D-glucose, though synthetically valuable, poses toxicity challenges .
Biological and Industrial Roles: Wine Industry: this compound and 4-O-acetyl-D-galacturonic acid coexist in grape metabolites but differ in roles; the former enhances mouthfeel, while the latter stabilizes color . Pharmaceutical Potential: 6-O-Galloylglucose’s antioxidant properties contrast with 6-chloro-6-deoxy-D-glucose’s role as a synthetic precursor .
Biological Activity
6-O-Acetyl-D-glucose is a derivative of D-glucose that has garnered attention for its potential biological activities. This compound is characterized by the presence of an acetyl group at the 6-position of the glucose molecule, which alters its chemical properties and biological interactions. Research has indicated that modifications at this position can influence various cellular processes, including insulin secretion, cell permeability, and cytotoxicity.
This compound is synthesized through acetylation of D-glucose, which typically involves the reaction of glucose with acetic anhydride or acetyl chloride in the presence of a base. The resulting compound retains the fundamental structure of glucose while introducing an acetyl group that can affect its solubility and reactivity.
Biological Activity
1. Insulin Secretion
Recent studies have shown that 6-O-acetyl derivatives can stimulate glucose-stimulated insulin secretion (GSIS) in pancreatic β-cells. For instance, a labdane-type diterpene containing 6-O-acetyl groups demonstrated significant stimulation of GSIS without cytotoxic effects in INS-1 rat pancreatic β-cells, suggesting potential therapeutic applications for managing type 2 diabetes .
2. Cytotoxicity and Cell Permeability
The introduction of an acetyl group at the C-6 position has been linked to altered cytotoxicity profiles in various cell lines. In one study, glycoconjugates modified at the C-6 position exhibited reduced toxicity to normal cells compared to those modified at the anomeric position. This suggests that C-6 substitution may enhance cellular uptake while minimizing adverse effects on healthy cells .
3. Apoptosis Induction
Research indicates that certain derivatives of this compound can induce apoptosis in cancer cell lines more effectively than their non-modified counterparts. For example, specific glycoconjugates demonstrated significant pro-apoptotic properties in HCT-116 and MCF-7 cancer cells, indicating their potential as anti-cancer agents .
Case Study 1: Insulin Secretion Enhancement
A study focused on a new labdane-type diterpene, which includes a 6-O-acetyl modification, showed that it significantly enhances GSIS in INS-1 cells. The compound's ability to promote insulin release without cytotoxicity positions it as a promising candidate for diabetes therapy .
Case Study 2: Reduced Cytotoxicity in Cancer Cells
In another investigation, derivatives of this compound were tested for their effects on cancer cell lines versus normal cells. The results indicated that these derivatives could selectively induce apoptosis in cancerous cells while preserving normal cell viability, highlighting their therapeutic potential with lower side effects .
Data Summary
Property | Observation |
---|---|
Insulin Secretion | Stimulated GSIS in INS-1 β-cells |
Cytotoxicity | Reduced toxicity to normal cells |
Apoptosis Induction | Induced apoptosis in cancer cell lines |
Cellular Uptake | Enhanced permeability via GLUT transport mechanisms |
Q & A
Basic Research Questions
Q. What are the common synthetic routes for regioselective acetylation of D-glucose at the 6-O position, and how do reaction conditions influence yield?
- Methodological Answer : The regioselective synthesis of 6-O-acetyl-D-glucose typically involves treating D-glucose with acetic acid (AcOH) under controlled conditions. For example, heating D-glucose in 50–75% aqueous AcOH at 100°C for 8–24 hours achieves moderate yields (10–30%) of the 6-O-acetylated product. Key variables include AcOH concentration, temperature, and reaction duration, which must be optimized to minimize byproducts like di-O-acetyl-D-glucose . Characterization via NMR or mass spectrometry is critical to confirm regioselectivity and purity.
Q. How can researchers validate the structural integrity of this compound post-synthesis?
- Methodological Answer : Nuclear magnetic resonance (NMR) spectroscopy is the gold standard for structural validation. For instance, -NMR can identify the acetyl proton signal at δ ~2.0–2.1 ppm, while -NMR confirms the acetyl carbonyl carbon at δ ~170–172 ppm. High-resolution mass spectrometry (HRMS) further verifies molecular weight accuracy. Cross-validation with enzymatic assays (e.g., acetate release quantification using kits like Megazymes’ Acetate-Kit) adds robustness .
Advanced Research Questions
Q. How do researchers resolve contradictions in reported acetylation yields across different synthesis protocols?
- Methodological Answer : Discrepancies in yields often stem from variations in reaction parameters (e.g., AcOH purity, heating uniformity, or glucose solubility). A systematic approach involves:
- Replicating protocols under identical conditions.
- Statistical analysis (e.g., ANOVA) to assess parameter significance.
- Byproduct profiling (e.g., HPLC or TLC) to track di-O-acetyl derivatives.
Studies suggest that anhydrous AcOH and precise temperature control (>100°C) improve reproducibility .
Q. What enzymatic assays are suitable for studying the metabolic or structural roles of this compound in biological systems?
- Methodological Answer : Marine Flavobacteria esterases (e.g., FI6_CE15) can hydrolyze 6-O-acetyl groups, releasing acetate, which is quantifiable via colorimetric assays (e.g., Megazymes’ Acetate-Kit). Concurrent NMR analysis of reaction mixtures validates specificity and kinetics. For in vivo studies, isotopic labeling (e.g., -glucose) paired with metabolic flux analysis tracks acetyl-group utilization .
Q. How can researchers optimize reaction conditions to suppress di-O-acetyl byproduct formation during synthesis?
- Methodological Answer : Di-O-acetyl byproducts arise from over-acetylation. Strategies include:
- Solvent modulation : Using polar aprotic solvents (e.g., DMF) to reduce AcOH reactivity.
- Temperature gradients : Gradual heating (e.g., 80°C → 100°C) to favor primary hydroxyl reactivity.
- Catalyst screening : Exploring non-acidic catalysts (e.g., DMAP) for milder conditions.
Post-synthesis purification via column chromatography (silica gel, ethyl acetate/hexane eluent) isolates the 6-O-acetyl product .
Q. Data Analysis & Experimental Design
Q. What statistical methods are recommended for analyzing variability in enzymatic hydrolysis data for this compound?
- Methodological Answer : For enzyme kinetics, use Michaelis-Menten models fitted via nonlinear regression (e.g., GraphPad Prism). Variability in triplicate assays can be assessed with coefficient of variation (CV) calculations. Outliers are identified using Grubbs’ test. For NMR data, principal component analysis (PCA) or multivariate analysis distinguishes spectral variations caused by impurities .
Q. How should researchers design experiments to investigate the stability of this compound under physiological conditions?
- Methodological Answer : Stability studies should simulate physiological pH (7.4), temperature (37°C), and ionic strength. Protocols include:
Properties
IUPAC Name |
[(2R,3R,4S,5R)-2,3,4,5-tetrahydroxy-6-oxohexyl] acetate | |
---|---|---|
Source | PubChem | |
URL | https://pubchem.ncbi.nlm.nih.gov | |
Description | Data deposited in or computed by PubChem | |
InChI |
InChI=1S/C8H14O7/c1-4(10)15-3-6(12)8(14)7(13)5(11)2-9/h2,5-8,11-14H,3H2,1H3/t5-,6+,7+,8+/m0/s1 | |
Source | PubChem | |
URL | https://pubchem.ncbi.nlm.nih.gov | |
Description | Data deposited in or computed by PubChem | |
InChI Key |
VFPUCPVAZOMVLI-LXGUWJNJSA-N | |
Source | PubChem | |
URL | https://pubchem.ncbi.nlm.nih.gov | |
Description | Data deposited in or computed by PubChem | |
Canonical SMILES |
CC(=O)OCC(C(C(C(C=O)O)O)O)O | |
Source | PubChem | |
URL | https://pubchem.ncbi.nlm.nih.gov | |
Description | Data deposited in or computed by PubChem | |
Isomeric SMILES |
CC(=O)OC[C@H]([C@H]([C@@H]([C@H](C=O)O)O)O)O | |
Source | PubChem | |
URL | https://pubchem.ncbi.nlm.nih.gov | |
Description | Data deposited in or computed by PubChem | |
Molecular Formula |
C8H14O7 | |
Source | PubChem | |
URL | https://pubchem.ncbi.nlm.nih.gov | |
Description | Data deposited in or computed by PubChem | |
DSSTOX Substance ID |
DTXSID70595099 | |
Record name | 6-O-Acetyl-D-glucose | |
Source | EPA DSSTox | |
URL | https://comptox.epa.gov/dashboard/DTXSID70595099 | |
Description | DSSTox provides a high quality public chemistry resource for supporting improved predictive toxicology. | |
Molecular Weight |
222.19 g/mol | |
Source | PubChem | |
URL | https://pubchem.ncbi.nlm.nih.gov | |
Description | Data deposited in or computed by PubChem | |
CAS No. |
7286-45-5 | |
Record name | 6-O-Acetyl-D-glucose | |
Source | EPA DSSTox | |
URL | https://comptox.epa.gov/dashboard/DTXSID70595099 | |
Description | DSSTox provides a high quality public chemistry resource for supporting improved predictive toxicology. | |
Disclaimer and Information on In-Vitro Research Products
Please be aware that all articles and product information presented on BenchChem are intended solely for informational purposes. The products available for purchase on BenchChem are specifically designed for in-vitro studies, which are conducted outside of living organisms. In-vitro studies, derived from the Latin term "in glass," involve experiments performed in controlled laboratory settings using cells or tissues. It is important to note that these products are not categorized as medicines or drugs, and they have not received approval from the FDA for the prevention, treatment, or cure of any medical condition, ailment, or disease. We must emphasize that any form of bodily introduction of these products into humans or animals is strictly prohibited by law. It is essential to adhere to these guidelines to ensure compliance with legal and ethical standards in research and experimentation.