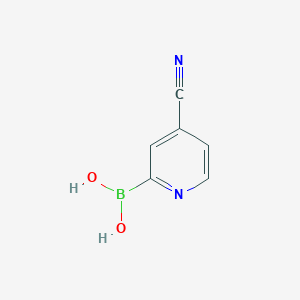
4-cyanopyridine-2-boronic acid
Vue d'ensemble
Description
Applications De Recherche Scientifique
4-cyanopyridine-2-boronic acid has numerous applications in scientific research:
Safety and Hazards
“(4-Cyanopyridin-2-yl)boronic acid” is considered hazardous according to the 2012 OSHA Hazard Communication Standard (29 CFR 1910.1200). It can be harmful by inhalation, in contact with skin, and if swallowed. It may cause skin corrosion/irritation, serious eye damage/eye irritation, and specific target organ toxicity (single exposure), with the respiratory system being a target organ .
Orientations Futures
The use of boron in the design of drugs is fairly recent and most biological activities of these compounds have been reported over the last decade . The introduction of boronic acid group to bioactive molecules has shown to modify selectivity, physicochemical, and pharmacokinetic characteristics, with the improvement of the already existing activities . This suggests that there is potential for future research and development of new drugs using boronic acids, including “(4-Cyanopyridin-2-yl)boronic acid”.
Mécanisme D'action
Target of Action
The primary target of 4-cyanopyridine-2-boronic acid is the Suzuki–Miyaura (SM) cross-coupling reaction . This reaction is a widely-applied transition metal catalysed carbon–carbon bond forming reaction .
Mode of Action
The SM coupling reaction conjoins chemically differentiated fragments that participate in electronically divergent processes with the metal catalyst . Oxidative addition occurs with formally electrophilic organic groups, whereby palladium becomes oxidized through its donation of electrons to form the new Pd–C bond . Transmetalation occurs with formally nucleophilic organic groups, which are transferred from boron to palladium .
Biochemical Pathways
The addition of B–H over an unsaturated bond occurs with syn-selectivity and proceeds in an anti-Markovnikov manner . Over-borylation of alkynes was problematic, but an oxidative dehydroborylation methodology employing a sacrificial aldehyde has since been found to readily revert back to the mono-borylated alkene . An observation that the reaction could be catalysed by ethers led to the development of new borane reagents .
Pharmacokinetics
The slow release rate of the active boronic acid allowed it to stay in low concentration, which led to a favourable partitioning between cross-coupling and oxidative homo-coupling . The low concentration of boronic acid also reduces the absolute rate of protodeboronation, which is highly useful for the coupling of unstable substrates .
Result of Action
The result of the action of this compound is the formation of a new carbon-carbon bond through the SM cross-coupling reaction . This reaction is mild and functional group tolerant, making it a valuable tool in organic synthesis .
Action Environment
The action of this compound is influenced by environmental factors such as the presence of ethers, which can catalyse the reaction . Additionally, the concentration of the active boronic acid in the reaction environment plays a crucial role in determining the outcome of the reaction .
Méthodes De Préparation
Synthetic Routes and Reaction Conditions: The synthesis of pyridinylboronic acids, including 4-cyanopyridine-2-boronic acid, can be achieved through several methods:
Metal-Halogen Exchange: This involves the reaction of pyridinyl halides with trialkylborates.
Metal-Hydrogen Exchange: This method uses substituted pyridine under specific conditions.
Industrial Production Methods: Industrial production methods for boronic acids often involve large-scale Suzuki-Miyaura coupling reactions, which are known for their mild and functional group-tolerant reaction conditions .
Analyse Des Réactions Chimiques
Types of Reactions: 4-cyanopyridine-2-boronic acid undergoes various chemical reactions, including:
Suzuki-Miyaura Coupling: This reaction forms carbon-carbon bonds between a substrate containing a halogen atom and the boronic acid in the presence of a palladium catalyst.
Oxidation and Reduction: These reactions can modify the functional groups attached to the pyridine ring.
Common Reagents and Conditions:
Palladium Catalysts: Used in Suzuki-Miyaura coupling reactions.
Oxidizing and Reducing Agents: Employed to alter the functional groups on the pyridine ring.
Major Products:
Carbon-Carbon Bonded Products: Resulting from Suzuki-Miyaura coupling.
Modified Pyridine Derivatives: From oxidation and reduction reactions.
Comparaison Avec Des Composés Similaires
Phenylboronic Acid: Another boronic acid used in Suzuki-Miyaura coupling.
Pyridinylboronic Acids: A class of compounds similar to 4-cyanopyridine-2-boronic acid.
Propriétés
IUPAC Name |
(4-cyanopyridin-2-yl)boronic acid | |
---|---|---|
Source | PubChem | |
URL | https://pubchem.ncbi.nlm.nih.gov | |
Description | Data deposited in or computed by PubChem | |
InChI |
InChI=1S/C6H5BN2O2/c8-4-5-1-2-9-6(3-5)7(10)11/h1-3,10-11H | |
Source | PubChem | |
URL | https://pubchem.ncbi.nlm.nih.gov | |
Description | Data deposited in or computed by PubChem | |
InChI Key |
XVCMGWIQWZNIRW-UHFFFAOYSA-N | |
Source | PubChem | |
URL | https://pubchem.ncbi.nlm.nih.gov | |
Description | Data deposited in or computed by PubChem | |
Canonical SMILES |
B(C1=NC=CC(=C1)C#N)(O)O | |
Source | PubChem | |
URL | https://pubchem.ncbi.nlm.nih.gov | |
Description | Data deposited in or computed by PubChem | |
Molecular Formula |
C6H5BN2O2 | |
Source | PubChem | |
URL | https://pubchem.ncbi.nlm.nih.gov | |
Description | Data deposited in or computed by PubChem | |
DSSTOX Substance ID |
DTXSID00674402 | |
Record name | (4-Cyanopyridin-2-yl)boronic acid | |
Source | EPA DSSTox | |
URL | https://comptox.epa.gov/dashboard/DTXSID00674402 | |
Description | DSSTox provides a high quality public chemistry resource for supporting improved predictive toxicology. | |
Molecular Weight |
147.93 g/mol | |
Source | PubChem | |
URL | https://pubchem.ncbi.nlm.nih.gov | |
Description | Data deposited in or computed by PubChem | |
CAS No. |
1072946-01-0 | |
Record name | (4-Cyanopyridin-2-yl)boronic acid | |
Source | EPA DSSTox | |
URL | https://comptox.epa.gov/dashboard/DTXSID00674402 | |
Description | DSSTox provides a high quality public chemistry resource for supporting improved predictive toxicology. | |
Retrosynthesis Analysis
AI-Powered Synthesis Planning: Our tool employs the Template_relevance Pistachio, Template_relevance Bkms_metabolic, Template_relevance Pistachio_ringbreaker, Template_relevance Reaxys, Template_relevance Reaxys_biocatalysis model, leveraging a vast database of chemical reactions to predict feasible synthetic routes.
One-Step Synthesis Focus: Specifically designed for one-step synthesis, it provides concise and direct routes for your target compounds, streamlining the synthesis process.
Accurate Predictions: Utilizing the extensive PISTACHIO, BKMS_METABOLIC, PISTACHIO_RINGBREAKER, REAXYS, REAXYS_BIOCATALYSIS database, our tool offers high-accuracy predictions, reflecting the latest in chemical research and data.
Strategy Settings
Precursor scoring | Relevance Heuristic |
---|---|
Min. plausibility | 0.01 |
Model | Template_relevance |
Template Set | Pistachio/Bkms_metabolic/Pistachio_ringbreaker/Reaxys/Reaxys_biocatalysis |
Top-N result to add to graph | 6 |
Feasible Synthetic Routes
Avertissement et informations sur les produits de recherche in vitro
Veuillez noter que tous les articles et informations sur les produits présentés sur BenchChem sont destinés uniquement à des fins informatives. Les produits disponibles à l'achat sur BenchChem sont spécifiquement conçus pour des études in vitro, qui sont réalisées en dehors des organismes vivants. Les études in vitro, dérivées du terme latin "in verre", impliquent des expériences réalisées dans des environnements de laboratoire contrôlés à l'aide de cellules ou de tissus. Il est important de noter que ces produits ne sont pas classés comme médicaments et n'ont pas reçu l'approbation de la FDA pour la prévention, le traitement ou la guérison de toute condition médicale, affection ou maladie. Nous devons souligner que toute forme d'introduction corporelle de ces produits chez les humains ou les animaux est strictement interdite par la loi. Il est essentiel de respecter ces directives pour assurer la conformité aux normes légales et éthiques en matière de recherche et d'expérimentation.